Determining the optimal transition to microfluidics
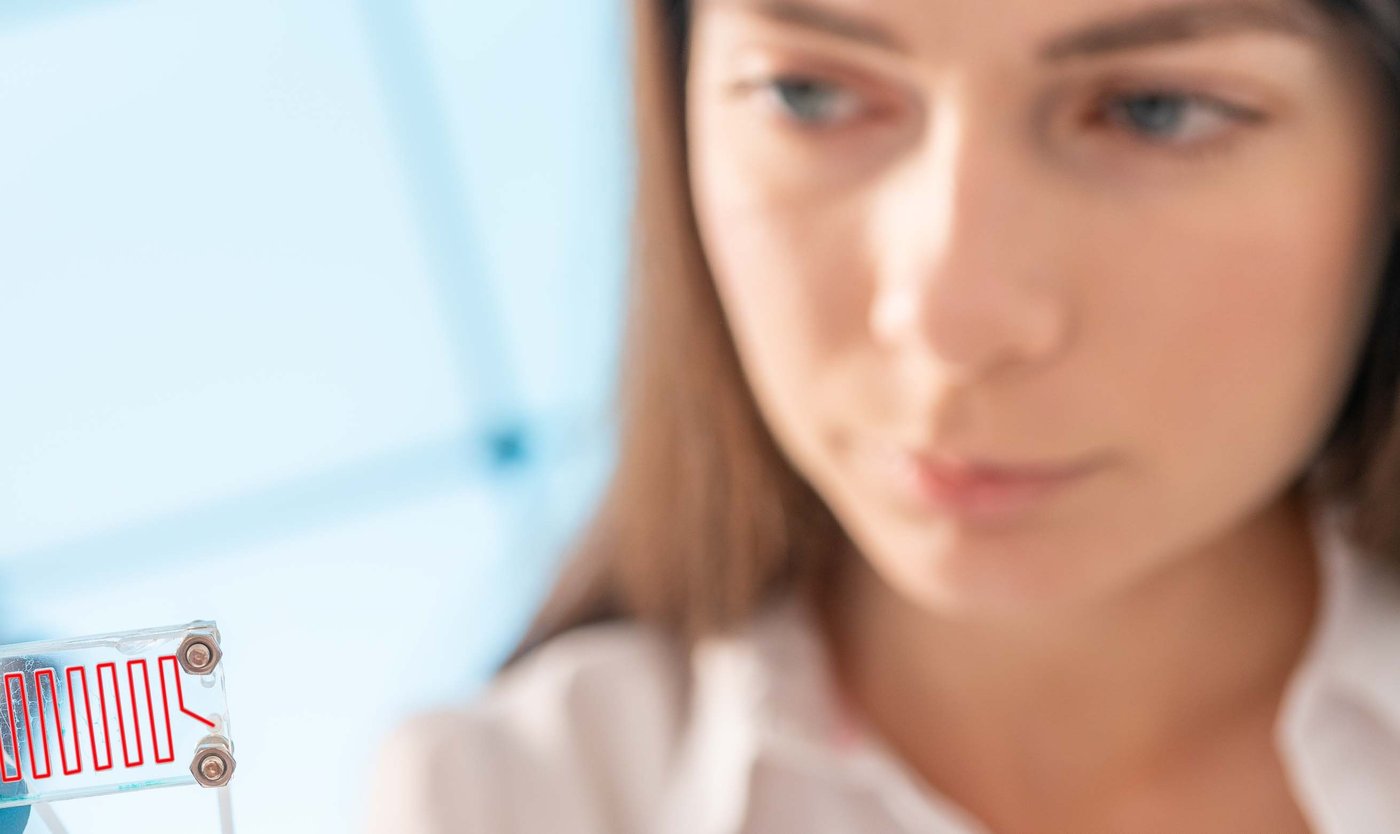
Posted by:
Hans-Jürgen Tiedtke
Published on:
Nov 22, 2022
Microfluidics is the key to high throughputs and low consumable costs. The biggest hurdle is frequently the transition between the inevitably macro-scale reagent tubes and the fine structures in the flow cells and cartridges. Finding the optimal solution requires not only extensive experience in a wide variety of applications, but also a deep understanding of molecular biology and laboratory processes, as HSE’s Head of Engineering Technologies, Hans-Jürgen Tiedtke, explains.
Mr. Tiedtke, you have been developing fluidics systems for many years. What is the biggest challenge with modern micro flow cells?
The biggest hurdle is often not – as you might think – construction of the small reaction cells. The most difficult problems arise at the transition between the reagents provided on a large scale and the reaction cells that have an extremely small volume. In extreme cases, the liquids must be taken from a milliliter vessel and transferred as volumes of a few microliters. By narrowing the diameters of the fluid channels, for example, either the pressure drop increases sharply or the flow rate is significantly slowed.
What are the most important economic constraints that impact problem solving?
The most important factors for users of automation solutions are time and reagent consumption. On the one hand, flushing very small channels can take a lot of time and, therefore, reduce the sample throughput of the device. On the other hand, the reagent costs can sometimes be very high. Therefore, it is often crucial to keep the tubes as short as possible and the flush volumes as small as possible.
You have found or analyzed very different solutions for this, from using geometry and capillary forces to mini-valves and finely drilled and milled plastic manifolds (see text boxes). Which criteria determine the best solution in each case?
The driver is always the application. The more closely the solution is tailored to the specific application, the more efficient the automation becomes. As an engineer, this requires you to understand as precisely as possible what the purpose of the flow cell and the entire device is in the context of the laboratory. For this, it is not enough to master only the engineering part. It also requires a deep understanding of the molecular biology that goes on in the reaction cells and of the entire laboratory process.
As a trained electrical engineer, how and where did you acquire this knowledge?
I worked on a hydrodynamic test system for artificial heart valves for my degree dissertation. As I joined QIAGEN in 2011 – and am therefore part of the original team that makes up HSE today – fluidic systems are virtually part of my everyday life. In the meantime, I have developed countless different applications. Your know-how grows with each new project. This is especially true when it comes to understanding the molecular biology that goes on inside the devices.
Can any engineer acquire this knowledge for the life sciences domain?
With time, you can certainly acquire a lot of knowledge about biology and chemistry. However, at HSE, there is an added feature that goes beyond personal experiential learning. We are not just engineers; we work together in multidisciplinary teams. In this way, I can also benefit directly from the knowledge and wealth of experience in biology and biochemistry of my colleagues – and they benefit likewise from my engineering expertise.
How does this multidisciplinary makeup of the development teams impact the project results?
The math is easy: The more experience there is from different disciplines and with different systems, the more conceptual options there are when finding solutions. And many pitfalls on the way to developing a productive device have already come to light by then. But the bottom line is that together we don’t just find more efficient solutions that better meet market needs. Thanks to our extensive experience, we also know what is important during development and this enables a device to be manufactured and operated as cost-effectively as possible.
Valve as close as possible to the flow cell connections
A flow cell used for drug screening must be connected to a total of 16 fluidic connections for filling and flushing. The tubes are conventional tubes with small diameters that draw reagents from storage vessels with volumes of up to one liter. The flow cell was designed to keep the connections permanently attached during the analysis process. To exchange the reagents in the flow cell as quickly as possible, a way of connecting the 16 tubes to the flow cell in a very confined space was needed, while positioning the valves that open and close the various reagent tubes as closely as possible to the cell. A special adapter head solved the problem by integrating all tubes. The adapter head can be positioned precisely on the cell.
Use geometry for fluid transport
To determine DNA concentrations in a spectrometer, a flow cell was designed so that the liquids move automatically through the channels into the measuring cell from a filling funnel using capillary action. As soon as the measuring cell is filled, the channel becomes wider and the capillary action stops. At this point, the funnel is also completely empty, so that only minimal evaporation can take place at the thin capillary cross-section. With this intelligent cell geometry, it was not just possible to elegantly solve precise dosing of the samples. At the same time, sample consumption could also be minimized.
Oil-water surface effects produce a thousand PCR reactions
Surface effects between oil and water were used to automatically generate the largest possible number of aqueous droplets in which PCR reactions can run in parallel. The aqueous solution containing all reagents for the PCR reaction is forced under pressure into the cavity between an oil film and a membrane. About a thousand droplets of the same size are produced, in which the PCR reaction is then carried out in parallel. Fluorescent makers enable visualization of positive results. If the initial concentration is selected smartly, the droplets that light up positively can be used, for example, to determine the exact virus concentration in a sample.
Moving the flow cell between connector groups
One way of making a reagent change as efficient as possible is to move the micro flow cell backwards and forwards between different connector groups. This method was selected for a next-generation sequencing device. The big advantage: Virtually no expensive reagents are wasted. The downside: A small air bubble forms with each change and must be flushed out.
Produce single cells via electromagnetic tapping
A fluidics system uses gravity and an electromagnetic tapping mechanism to separate individual cells and place them in microtiter plates. The channel through which the cell suspensions are filled tapers into a narrow silicon channel that is open at the bottom. An electromagnetic force is triggered to tap a silicon membrane, which enables droplets of the same size to be produced at the outlet. Using a camera, drops containing one, several or no cells can then be automatically differentiated and positioned accordingly.
A valve for complex sample-to-result cartidge
To carry out complete sample-to-result PCR reactions, including DNA purification and concentration in a cartridge, a valve needs to be developed that enables specific liquid change. The solution was a small rubber part that can be closed and opened by a certain force using a miniature plunger. The second challenge was the large sample volumes that needed to be concentrated for the analysis. To fill them directly using a pipetting robot, a funnel was designed in such a way that it can be hermetically closed via pressure through the pipette tip. Thus, the sample can be easily injected.
Manifold allows for short distances and flush times
The number of bacteria in a liquid can be accurately determined by the change in electrical impedance in a measurement channel. However, this requires the sample liquid to be forced through a measurement channel of only 15 micrometers. Even at high pressures, flow rates of just 30 µl/min are achieved. To minimize the time needed to clean the system before the next measurement, it is essential that the tubes to the flow cell are kept as short as possible and have a small internal diameter. Thanks to a so-called manifold, the additional volumes of liquid required in the tubes were limited to a few microliters. The channels, which are only 500 micrometers in diameter, were milled and drilled for this purpose in two plastic plates, which were then joined using a bonding process to form the functional manifold channel system.